Fermilab scientist Wes Ketchum discusses the mysteries of neutrinos
Wes Ketchum discussing neutrinos
April 20, 2018
If you hold up your hand towards the light of the sun, every second, 65 billion subatomic particles called neutrinos pass through the fingernail on your thumb and continue on their near-speed of light voyage across the universe.
These mysterious particles behave unlike any others known to physics and may hold the answers to the questions perplexing scientists about the fundamental nature of our universe.
In an attempt to discern their complexities, scientists have burrowed into the South Pole distributing sensors in Antarctica’s purest ice at depths between 1,450 to 2,450 meters (the Eiffel Tower is 324 m tall), with the aptly named IceCube Neutrino Observatory. IceCube’s neutrino detector is made of 1.5-2.5 cubic kilometers of pure ice. That would be enough water to fill one million Olympic-sized swimming pools.
Scientists have also tunneled 3,300 feet into an abandoned mine drilled beneath Japan’s Mount Ikeno. The Super-Kamiokande Neutrino Detection Experiment placed a cylindrical tank 131 feet in height, holding 50,000 tons of ultrapure water with 13,000 hand-blown glass light detectors in an attempt to capture these tricky particles that hardly interact with normal matter and usually pass straight through anything in its path.
To discuss their perplexities and the fascinating secrets their mysteries might hold, College of DuPage hosted a free Steminar on April 6th open to the public, featuring speaker Wes Ketchum. He is an associate scientist at Fermi National Accelerator Laboratory (Fermilab) in Batavia and a member of the MicroBooNE collaborate at Fermilab researching neutrinos. He has spent the last year working at the European Organization for Nuclear Research (CERN) in Switzerland.
Fermilab has operated neutrino-producing facilities for more than 30 years.
Neutrinos are elementary particles in the Standard Model of physics, meaning they cannot be broken down into smaller parts. They are created through the process of radioactive decay; whether it’s the beta decay of atomic nuclei or hadrons, nuclear reactions such as those that take place in the core of a star, or in artificial nuclear reactors, including nuclear bombs and particle accelerators. Some supernovae lose more than 99% of their energy through neutrino release.
The majority of neutrinos in and around the Earth, are as a result of nuclear reactions from our Sun. The Sun produces 70 billion electron neutrinos per cm2 per second. Next to photons, neutrinos are the second most abundant particle in the universe. However, this abundance hasn’t made neutrinos any easier to detect.
Unlike other known particles, neutrinos do not participate in the strong interaction (fundamental force binding matter together) and therefore travel through most matter unimpeded at a little less than the speed of light. They can even travel directly through black holes. They only interact via the weak subatomic force (governs particle decay). Unlike other leptons such as the electron, they are considered neutral particles because they don’t possess an electrical charge.
Due to their nature of passing straight through most matter without any reactions, neutrinos are incredibly hard to observe. This is why scientists must build enormous and outlandish detectors just to observe a few interactions.
Ketchum explained, “The typical neutrino would travel through more than a light-year of lead before interacting.” Lead is incredibly dense and is used in x-ray imaging to protect us from radiation. From here to the sun is only a distance of eight light minutes. This has inspired scientists to come up with ambitious solutions and undertake audacious engineering projects.
Ketchum describes the humorous story how in 1951 scientists Frederick Reines and Clyde Cowan spurred on by the atomic weapon studies in the Manhattan Project schemed up direct detection of neutrinos. They planned to set off nuclear bombs with detectors nearby to observe neutrinos. When the lunacy of their plan was pointed out, they compromised, placing detectors near the Savannah River nuclear reactor successfully observing neutrinos in 1956.
Further outlandish attempts were undertaken by Ray Davis in 1966, who placed over 100,000 gallons of dry-cleaner fluid a mile beneath ground at the Savannah River nuclear reactor collecting only one neutrino detection every two days. This was considered a resounding success.
Their overly-ambitious engineering was worth the efforts because the Standard Model leaves some physical phenomenon unexplained. It fails to describe a complete theory of fundamental interactions.
Ketchum explained how the Standard Model fails to accommodate for neutrino oscillation, a phenomenon where a neutrino created with a distinct lepton flavour (electron, muon, or tau) can later be observed to possess a different flavour. Neutrinos can change their flavours in flight, each maintaining a different mass.
For example, an electron neutrino produced in a beta decay reaction may interact in a distant detector as a muon or tau neutrino. When they interact with atoms in detectors they produce different end products that correlate to their flavour name.
For the longest time, neutrinos were predicted erroneously by the Standard Model to contain no mass. However, oscillation requires mass to differentiate between them. This discovery led to the 2015 Nobel Prize for physics for the Sudbury Neutrino Observatory.
Neutrinos have an infinitesimally small mass compared to other known particles. They weigh at least a million times less than an electron, the lightest other known particle.
However, keeping with their mysterious nature, each flavour does not have a directly corresponding mass, but rather a unique mix of the possible masses. Ketchum explained this is because quantum mechanics tells us particles are not definite entities but uncertain bundles of probability.
Ketchum propositions, since the Standard Model predicts neutrinos should be massless, what new phenomenon gives them mass.They don’t acquire mass the way other particles do, interacting with the Higgs Field.
As far as physicists know, neutrinos are the only particles that undergo oscillation. Including these three flavours, for each neutrino there also exists a corresponding antiparticle called an antineutrino.
Theories for how neutrinos possess mass state they could either be their own antiparticles, or perhaps they are interacting with a new, undiscovered field that gives them mass. This would require the discovery of new neutrinos, and leads to the question of whether this new field affects all other known particles as well.
Ketchum explained to test these theories and better understand neutrino oscillation, Fermilab is creating the $1.5 billion Deep Underground Neutrino Experiment (DUNE).
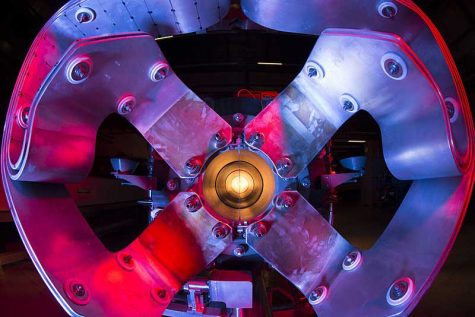
Fermilab NuMI focusing horn for creating neutrino beams
A new accelerator at Fermilab will accelerate protons into graphite creating beams of neutrinos. These neutrinos will then travel through 1,300 kilometers of earth’s soil from Batavia to a massive underground detector at Lead, S.D. The detector will be placed in an abandoned underground mine, shielding their observations from the polluting cosmic rays.
This extremely long distance gives scientists the greatest possibility to observe neutrino oscillation in all of its forms.
DUNE is the most ambitious particle physics experiment to be attempted in the US since the failed Superconducting Super Collider in the 90’s. The project involves 1,000 researchers from over 30 countries.
The far detector in S.D. has four modules, each containing 17,000 metric tons of liquid argon. If a neutrino strikes an argon atom, it produces particles such as electrons and photons that the detector can observe. For the projects immensity, they are still expecting to only observe 10 to 20 neutrinos per day.
Ketchum hopes the experiment will distinguish whether the three flavours come in a normal hierarchy (2 lightweight and 1 heavy flavour) or inverted hierarchy (2 heavy and 1 lightweight flavour).
Ketchum explained what particularly excites him saying, ”Modern physics does not contain an explanation of the imbalance between matter and antimatter, answering questions such as why does life even exist. Now with this new window of neutrino oscillations, this asymmetry could be accommodated for.”
Cosmologists predict after the Big Bang, both antimatter and matter should have existed in equal shares, annihilating each other. However, what gave rise to the imbalance?
To account for this asymmetry, scientists are looking for a type of particle that behaves differently from its antimatter counterpart. The question becomes do antineutrinos oscillate from flavour to flavour at different rates than neutrinos?
Ketchum strives on searching for other kinds of neutrinos, using Fermilab’s Short Baseline Neutrino Oscillation experiment.
He hopes his endeavours will one-day shed light upon the mysteries perplexing the Standard Model and why we live in a universe of matter, where galaxies formed, stars shine, and life has the opportunity to flourish.
*for more on DUNE visit Fermilab’s youtube video The Science of the Deep Underground Neutrino Experiment (DUNE)
*the next STEMinat at COD: Thursday, April 12, 2018 at 4 p.m.
Dr. Mark SubbaRao, Adler Planetarium
“Advancing Education and Research through Immersive Data Visualization”